Sunday, June 17, 2018
Musean Hypernumbers
By
CNu
at
June 17, 2018
0
comments
Labels: awareness , fractal unfolding , implicate order , neuromancy , quorum sensing? , subrealist oeuvre... , visitors? , work
Friday, June 15, 2018
Are Space And Time Quantized?
By
CNu
at
June 15, 2018
0
comments
Labels: awareness , fractal unfolding , implicate order , neuromancy , quorum sensing? , subrealist oeuvre... , visitors? , work
Wednesday, May 16, 2018
DIY DNA Tinkering...,
By
CNu
at
May 16, 2018
0
comments
Labels: fractal unfolding , gain of function , Genetic Omni Determinism GOD , human experimentation , information anarchy , Malnare , Metagenomics , Noo/Nano/Geno/Thermo
Wednesday, October 04, 2017
Access the Guardian Through a Raspberry Pi? Of Course...,
An Introduction to the Wolfram Language Online
By
CNu
at
October 04, 2017
0
comments
Labels: AI , comedy gold , Exponential Upside , fractal unfolding , priceless....
A New Kind of Science
Simple programs
- Its operation can be completely explained by a simple graphical illustration.
- It can be completely explained in a few sentences of human language.
- It can be implemented in a computer language using just a few lines of code.
- The number of its possible variations is small enough so that all of them can be computed.
Mapping and mining the computational universe
By
CNu
at
October 04, 2017
0
comments
Labels: AI , Exponential Upside , fractal unfolding
Sunday, October 01, 2017
Quantum Criticality in Living Systems
By
CNu
at
October 01, 2017
0
comments
Labels: AI , fractal unfolding , gain of function , Genetic Omni Determinism GOD , quantum , transbiological , What IT DO Shawty...
Friday, September 29, 2017
Why the Future Doesn't Need Us
By
CNu
at
September 29, 2017
0
comments
Labels: AI , azoth , count zero , evolution , Exponential Upside , fractal unfolding , gain of function , implicate order , People Centric Leadership , scientific morality , Slice vs. Proprietors , Small Minority
Thursday, September 28, 2017
Poised Realm Patent
- [0001]This application claims the benefit of U.S. Provisional Application Nos. 61/367,781, filed Jul. 26, 2010; 61/367,779, filed Jul. 26, 2010; 61/416,723, filed Nov. 23, 2010; 61/420,720, filed Dec. 7, 2010; and 61/431,420, filed Jan. 10, 2011, all of which are incorporated herein by reference in their entirety.
BACKGROUND
- [0002]1. Field of the Invention
- [0003]The present invention relates to systems and uses of systems operating between fully quantum coherent and fully classical states. Non-limiting applications include drug discovery, computers, and artificial intelligence.
- [0004]2. Background Description
- [0005]Many physical systems having quantum degrees of freedom quickly decohere to classicity for all practical purposes. Thus, many designed systems consider only classical behaviors. One example is in the field of drug discovery where traditional approaches to drug design considers the lock-and-key fitting of a molecule into an enzyme or receptor. Other designed systems are carefully setup to maintain full quantum coherence, for example, the qubits in a quantum computer. However, recent discoveries have indicated several systems in nature that have relatively slow decoherence. Birds are able to see magnetic field lines due to a quantum coherent chemical reaction in their retina. Light harvesting molecules are able to maintain quantum coherent electron transport for times much longer than the expected coherence time at room temperatures. The existence of such cases demonstrates that quantum coherence can exist at room temperature and at the presence of water bath and evolution can ‘design’ quantum coherent structures to play certain biological roles. Thus, there is a need for new systems that utilize the unique properties that exist between full quantum coherence and classicitySUMMARY OF THE INVENTION
- [0006]Disclosed herein are various methods of classifying the state of a system, such as a molecule interacting with its environment, in terms of its degree of order, its degree of coherence, and/or its rate of coherence decay. Some embodiments include classifying only a single one of these variables whereas other embodiments include classifying two or all three of the variables. These methods include classifying the system in the course of creating systems that exist and/or operate at a specific point or region of a classification space described the variables discussed above and all practical outcomes of such creation.
- [0007]Disclosed herein is a quantum reservoir computer that includes a plurality of nodes, each node comprising at least one quantum degree of freedom that is coupled to at least one quantum degree of freedom in each other node; at least one input signal generator configured to produce at least one time-varying input signal that couples to the quantum degree of freedom; and a detector configured to receive a plurality of time-varying output signals that couple to the quantum degree of freedom.
- [0008]Also disclosed herein is a method of drug discovery that includes selecting a biological target; screening a library of candidate molecules to identify a first subset of candidate molecules that bind to the biological target; determining the energy level spacing distribution of a quantum degree of freedom in each of the candidate molecules in the first subset; comparing the energy level spacing distribution to at least one pre-determined reference function; and selecting a second subset of molecules from the first subset as drug candidates based on the comparison.
- 0009]Further disclosed herein is a method of drug discovery that includes selecting a biological target; screening a library of candidate molecules to identify a first subset of candidate molecules that bind to the biological target; determining the energy level spacing distribution of a quantum degree of freedom in each of the candidate molecules in the first subset; conducting an in vitro or in vivo assay for biological activity on each of the candidate molecules in the first subset; correlating the energy level spacing distribution with activity determined from the in vitro or in vivo assay; determining the energy level spacing distributions of a quantum degree of freedom in a new set of candidate molecules; comparing the energy level spacing distributions of the new set of candidate molecules with energy level spacing distributions that correlate with biological activity; and select as drug candidates from the new set of candidate molecules those molecules whose energy level spacing distributions exhibit a pre-determined level of similarity to the energy level spacing distributions that correlate with biological activity.
- [0010]Further disclosed herein is a method of drug discovery that includes selecting a biological target; screening a library of candidate molecules to identify a first subset of candidate molecules that bind to the biological target; measuring decoherence decay of a quantum degree of freedom in each of the candidate molecules in the first subset; comparing the decoherence decay to at least one pre-determined reference function; and selecting a second subset of molecules from the first subset as drug candidates based on the comparison.
-
By
CNu
at
September 28, 2017
0
comments
Labels: as above-so below , azoth , Exponential Upside , fractal unfolding , What IT DO Shawty...
Sunday, December 11, 2016
the semiosis of evolution
By
CNu
at
December 11, 2016
0
comments
Labels: fractal unfolding , Genetic Omni Determinism GOD , gestalt , human experimentation , implicate order , macrobiology
Friday, October 28, 2016
Computational Genomics F'Real...,
By
CNu
at
October 28, 2016
0
comments
Labels: fractal unfolding , Genetic Omni Determinism GOD , transbiological , What Now?
Tuesday, June 07, 2016
quantum criticality at the origin of life
By
CNu
at
June 07, 2016
0
comments
Labels: fractal unfolding , quantum , What IT DO Shawty...
Tuesday, November 24, 2015
control of fractal unfolding - impressive, most impressive...
By
Dale Asberry
at
November 24, 2015
0
comments
Labels: as above-so below , Childhood's End , evolution , fractal unfolding , gain of function , Genetic Omni Determinism GOD , What IT DO Shawty
Fuck Robert Kagan And Would He Please Now Just Go Quietly Burn In Hell?
politico | The Washington Post on Friday announced it will no longer endorse presidential candidates, breaking decades of tradition in a...
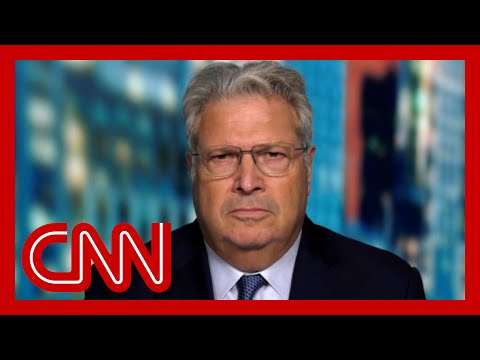
-
theatlantic | The Ku Klux Klan, Ronald Reagan, and, for most of its history, the NRA all worked to control guns. The Founding Fathers...
-
Video - John Marco Allegro in an interview with Van Kooten & De Bie. TSMATC | Describing the growth of the mushroom ( boletos), P...
-
dailybeast | Of all the problems in America today, none is both as obvious and as overlooked as the colossal human catastrophe that is our...